1. Subject recruitment
- Subject population
- Subjects are recruited by advertisement to fit the specific demographics required for the study.
- The subject for this particular study is a healthy, nonsmoker between the ages of 19 - 45, with no history of heart or lung disease.
- Informed consent
- This study is approved by the University of California, San Diego, Human Research Protections Program.
- The subject is informed of the potential risks of this study, e. g. magnetic field exposure (MRI) and of breathing hypoxic and hyperoxic gas.
If the subject is female and capable of childbearing, a urine sample is collected before the onset of the study in order to rule out pregnancy. Although exposure to magnetic fields is extremely unlikely to be harmful to a developing fetus, the exact potential risk is unknown. In addition hypoxia might present a risk to the fetus. For these reasons pregnant women are excluded from the study.
- MR safety screening
All our subjects must complete a questionnaire about items that would contraindicate a magnetic resonance examination. If a contraindication is found, the subject is excluded from the study.
2. Preparation
- Physical examination
- The subject is interviewed regarding their physical health and habits, and receives a brief physical examination by a licensed physician.
- The subject's height and weight are also measured. These values are used to estimate the predicted values of pulmonary function test and the specific absorption rate (SAR). The estimation of SAR is important because there is the potential for heating of the subject's tissue from the radio frequency (RF) energy necessary to produce the MR images.
- Pulmonary function test
Lung volumes are measured using spirometry in the upright posture. The subject performs pulmonary function testing by blowing into a handheld spirometer (EasyOne Spirometer, Medical Technologies Andover). A nose clip is used to ensure all air is expelled out of the mouth. Spirometry data are acquired in triplicate to ensure reliable data. The quality of the pulmonary function test must meet the American Thoracic Society / European Respiratory Society criteria5.
3. Undergoing the magnetic resonance study
- Training session to produce a reliable functional residual capacity (FRC) lung volume during breath holding.
Our lung images are acquired when the lung is at FRC. This is an easily attainable lung volume, and enhances signal to noise in the image, by increasing the proton density. Our subjects are trained to comfortably reproduce this lung volume. If the subject is a first-time participant in one of our studies, this training session is completed before the subject enters the MR scanner. Since the scanner makes sounds during acquisition, a sound recording of the image acquisition is played for the subject, and the subject is coached while they practice synchronizing their breathing with the sound recording.
- Breathing setup
- The inspiratory setup
The inspiratory tube is connected to the Mylar gas bags, which will hold either hyperoxic and hypoxic gases, or normoxic air, and are administered via a switching valve (Single-Piston Sliding-Type™ valve and controller 4285A, Hans Rudolph). The valve is checked for normal functioning.
The gas bags, which administer the inspired gases to the subject, are in the scanner room and are connected to gas tanks of different oxygen concentrations in the MR console room. Gas is added to the bag by the investigator through manipulation of the gas tank regulators.
The investigator must monitor the bag through the console room window to ensure the gas volume is sufficient, in order to ensure that the subject has enough gas to inspire normally. The FIO2 of hyperoxic and hypoxic gases are 1.0 and 0.125, respectively. Room air is used for the normoxic gas.
- The expiratory setup
The expiratory breathing tube is sufficiently long to connect from the subject in the MR scanner through a pass-through to the metabolic cart (TrueOne 2400, ParvoMedics) in the MR console room.
The metabolic cart measures the volume of expired air as well as mixed expiratory O2 and CO2 concentrations. Based on these parameters, it also calculates various respiratory volumes, such as tidal volume, oxygen consumption (V. O2), carbon dioxide production (V. CO2), and respiratory quotient.
It is necessary to calibrate the O2 and CO2 sensors and flow meter before every study. An investigator calibrates the metabolic cart system by following the built-in instructions in accordance with the metabolic cart operating software. O2 and CO2 sensors are adjusted by two points calibration between the calibration gas (FO2 = 0.16 and FCO2 = 0.04) and room air (FO2 = 0.2098 and FCO2 = 0.00). The flow meter is calibrated by using a standardized 3-liter syringe. The investigator pumps 3-liter volumes of air (at room temperature and barometric pressure) into the metabolic cart through the expiratory breathing tube equipped with a Hans Rudolph 2-way non-rebreathing valve which is identical to the one that is attached to the subject’s mask. In order to allow for the various flow rates of breathing, this calibration is performed at least five times with peak flows ranging from 50 L/min ATPS to 80 L/min for resting measurements. The volume in the tubing and mouthpiece is calculated and when combined with the subjects respiratory data this allows for correction of the delay time caused by the length of expiratory tubing.
- Mask
A cold-sterilized silicone mask (7400 series Oro-Nasal Mask, Hans Rudolph) is fitted to the subject to allow delivery of different gas mixtures and acquisition of metabolic and ventilatory data throughout the imaging session (size: petite, small, medium, large and extra large). A majority of adult subjects are fitted with small, medium or large masks.
The mask equipped with a pre-sterilized non-rebreathing valve (Two-way non-rebreathing valve T-Shape™ configuration, 2600 Medium, 2700 Large, Hans Rudolph) is fixed on the subject’s face with a mesh attachment and checked for leaks. Then, inspiratory and expiratory tubes are attached.
- MR setup
- The subject lies supine, with feet towards the scanner bore on a sliding table that moves into the magnetic resonance scanner.
- Pillows and foam pads are used to maximize the subject’s comfort. A pulse oximeter (7500 FO, Nonin) is placed on the subject’s finger to monitor oxygen saturation and heart rate, which is especially important when the subject is exposed to hypoxia.
- An EKG electrode pad is placed on the subject’s chest. This allows the arterial spin labeling (ASL) MR sequence to be gated to the QRS complex.
- Once the subject is wearing a mask they cannot easily communicate with the study personnel. A squeeze ball is positioned in the subject’s hand, and taped in place. This allows for the subject to alert the investigators at any time that they need assistance.
- Earplugs are given to the subject to protect them from the noise produced by the scanner.
- Three MR phantoms are placed on the subject’s chest. The phantoms are used to quantify the MR signal during the post processing and have previously been characterized.
- The torso coil is also placed over the phantoms on the subject’s chest. The torso coil is used to increase the signal to noise ratio of MR image compared with the body coil by reducing the physical distance between the receiver and the subject. Finally, the subject is covered with a blanket to ensure their comfort.
4. MR scanning
- Before scanning
- The subject is asked to lie feet first on the MRI scan table. Then the scan table moves the subject into the center of the MRI scanner bore.
- The scanner operator frequently talks to the subject in order to make sure that the subject is comfortable and to remind them to squeeze the squeeze ball if they need assistance.
- Investigators monitor the EKG, O2 saturation, tidal volume, V. O2 and V. CO2. The first few minutes of monitoring are especially important to ensuring good quality data; if these numbers are not in the expected range, the calibration must be repeated and the facemask and tubing checked for leaks.
- Imaging exam sequences
- The localizer sequence is acquired first to obtain the anatomical images to determine placement of the imaging slice within the torso.
- An slice is selected in the sagittal plane from the portion of the right lung where the anterior – posterior distance is the largest. The slice thickness is typically 15 mm and a field of view is 40 cm x 40 cm.
- Arterial spin labeling
Arterial spin labeling - flow-sensitive alternating inversion recovery with an extra RF pulse sequence (ASL- FAIRER) with a half-Fourier acquisition single-shot turbo spin-echo (HASTE) imaging scheme is used to obtain the regional perfusion data 1,2.
- The subject will hear a series of sound pairs, “bang-bang” indicating the magnetic tag and the image acquisition. The first banging sound is shorter than the second. This difference in the sound is noticeable. In between these sound pairs, the subject must complete one breathing cycle: breath-in and breath-out, prior to the next pair. During the pairs of sounds, the subject must breathhold at FRC.
- The subject is given a test run of image acquisitions during which time the subjects practices the breathing that they were familiarized with before they were put into the scanner (described above).
- The MR operator evaluates the quality of lung images based on the movement of the diaphragm. If the movement is minimal the ASL measurements start. The investigators monitor the tidal volume. The approximate target tidal volume is 500-700 ml consistent with normal ventilation with additional deadspace from the valve.
- The basic principle to quantify the pulmonary perfusion is described in reference 1 and 2 in detail. In this MR sequence, two different cardiac-gated images are acquired with a 5 second interval in between them. The image timing between the tag and the image acquisition (i.e. between the first bang sound and second bang sound) is set to 80% of the R-R interval to allow for the collection of one systolic ejection of blood. The signal from the blood is prepared in two different ways. In one image, the longitudinal magnetization of both blood and tissue inside and outside of the image slice is inverted, resulting in very low signal from both blood and tissue. In the second image, the inversion is applied only to the imaged slice, with the result that the inflow of blood from outside the image slice into the slice has a strong MR signal. When the two images are subtracted, thereby canceling out the stationary signal, the result is a quantitative map of blood delivered to the image slice within one systolic ejection period. The resolution is 256 x 128 pixels; therefore, the voxel size is ˜1.5 x ˜3.1x 15 mm (˜0.07 cm3).
- Lung proton density
In addition to the ASL images, we also use a multi-echo fast gradient echo (mGRE) sequence to measure lung proton density 3. This allows the perfusion measurements to be expressed in mL/min/g and accounts for lung tissue deformation inside the thorax6. This sequence is run twice, one for the torso coil and one for the body coil.
- During this proton density image acquisition, the subject will hear a continuous noise that will last for approximately 10 seconds. During this, the subject must hold their breath and stay at FRC.
The basic principle to quantify the lung proton density is described in reference 3. The resolution is 64 x 64 pixels; therefore, the voxel size is ˜6.3 x ˜6.3 x 15 mm (˜0.59 cm3).
- Switching inspiratory gases
- In this study gases with FIO2 = 0.21 (normoxia/room air), FIO2 = 0.125 (hypoxia), and FIO2 = 1.00 (hyperoxia) are presented in balanced order between subjects, although these can be varied as desired, consistent with the research goals
- After a subject reaches steady state for a specified condition (˜20 minutes for a particular gas) 7, MR measurements of perfusion and proton density are acquired. In this case, the 20 minute period of exposure to the gas before imaging is chosen because although the initiation of hypoxic pulmonary vasoconstriction response occurs within seconds, the response to alveolar hypoxia is not maximal until ˜20 minutes 8, consistent with the goal of this particular study.
5. Post processing
Post processing is completed using custom developed software within the MATLAB programming environment.
- Coil inhomogeneity correction
By using the paired mGRE images from the homogeneous body coil and the inhomogeneous torso coil (section 4.1), all blood flow and proton density images are corrected for coil inhomogeneity on a subject-by subject basis 6. This method is described in reference 6 and 9.
- Density normalized perfusion
- Once the subtracted ASL image is corrected for coil inhomogeneity, the regional pulmonary blood flow is quantified in milliliters (blood) per minute per cubic centimeter (voxel).
- Density normalized perfusion expressed in units of milliliters (blood) per minute per gram of water is calculated by dividing the ASL image by the proton density image to give perfusion in milliliters per minute per gram of lung (tissue + blood).
- A mutual information based technique that includes translation and rotation is utilized to register the ASL and proton density images, and the ASL perfusion image is divided by the proton density image obtained with the torso coil on a voxel by voxel basis 6,9. This method is also described in reference 6 and 9.
- Data analysis
For each image acquired as described above (lung proton density and density normalized perfusion), the data are analyzed in the following manner.
- For each image, mean density-normalized perfusion is calculated.
- Three different indexes of perfusion heterogeneity are calculated. These are 1) relative dispersion4,10,11, also known as the coefficient of variation, a global scale of heterogeneity defined as the ratio of the standard deviation to the mean perfusion in which the larger the relative dispersion, the more heterogeneous the perfusion distribution; 2) fractal dimension (Ds) 7, an index of the spatial heterogeneity that is scale independent, where the value varies between 1.0 (homogeneous) and 1.5 (spatially random); and 3) a geometric standard deviation, also global scales of heterogeneity but based on log normal model distribution 2.
6. Representative results
Physiological data are given in Table 1. Heart rate was increased in hypoxia and saturation was decreased. Ventilation was 8.31 L/min BTPS during hypoxia, 7.04 L/min during normoxia, and 6.64 L/min during hyperoxia. Tidal volume was 0.72 L during hypoxia, 0.69 L during normoxia, and 0.67 L during hyperoxia. The exposure to hypoxia increases both ventilation and tidal volume, while the hyperoxia decreases ventilation and tidal volume.
Three density normalized perfusion images collected during the three different inspired oxygen concentrations (Hypoxia: 0.125, Normoxia: 0.21, and Hyperoxia: 1.00) obtained from one subject (male, 30 years of age) are shown in Figure 1. The results of the data analysis of perfusion heterogeneity are given in Table 2. It can be seen that hypoxia increased the relative dispersion however the other indices were largely unchanged.
Figure 2 shows the effect of inspired oxygen concentrations on the vertical distribution of density normalized perfusion,
averaged every 1 cm below 10 cm height from the most dependent part of lung and above 10 cm. All data points above 10 cm are averaged and
displayed as one data point.
| Hypoxia | Normoxia | Hyperoxia |
Heart Rate (bpm) | 60 | 51 | 50 |
SpO2 | 86 | 99 | 100 |
VE BTPS (L/min) | 8.31 | 7.04 | 6.64 |
Vt BTPS (L) | 0.76 | 0.69 | 0.67 |
FEO2 (%) | 8.85 | 17.27 | - |
FECO2 (%) | 3.41 | 3.60 | 3.20 |
VO2 STPD (L/min) | 0.25 | 0.22 | -* |
VCO2 STPD (L/min) | 0.23 | 0.21 | 0.18 |
Table 1. The physiological data during scanning session.
* When the subject is breathing 100% oxygen, VO2 cannot be easily measured (see 12for details).
| Hypoxia | Normoxia | Hyperoxia |
Relative Dispersion | 1.07 | 0.85 | 0.87 |
Fractal Dimension | 1.24 | 1.26 | 1.26 |
Geometric Standard Deviation | 2.41 | 2.11 | 2.38 |
Table 2. The three indices of pulmonary perfusion heterogeneity.
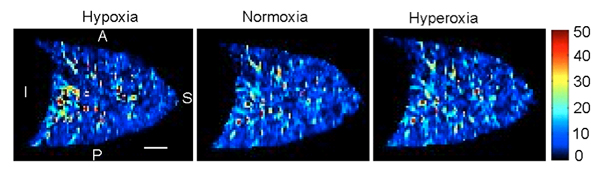
Figure 1. Effect of three different inspired oxygen concentrations on density normalized perfusion. 1.1: Hypoxia (0.125), 1.2: Normoxia (0.21), 1.3: Hyperoxia (1.00). The scale is 3 cm (white solid line). A: anterior, P: posterior, I: inferior, and S: superior directions, respectively.
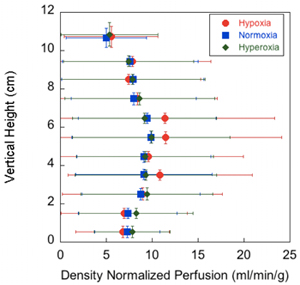
Figure 2. Effect of three different inspired oxygen concentrations on the vertical distribution of density normalized perfusion. The density normalized perfusion is averaged within 1 cm bins in the same gravitational plane, starting from 0 cm at the most dependent part of the lung and continuing to the most nondependent portion. All data points above 10 cm are averaged and displayed as one data point.
The error bars represent the standard deviation of the values of the density normalized perfusion within that plane. Hypoxic data are in red, normoxic data are in blue, and hyperoxic data are in green.